Cancer is challenging to cure due to its complexity and variability. It involves numerous types of diseases, each with different characteristics, genetic mutations, and responses to treatment. Cancer cells can adapt, evolve, and develop resistance to therapies, making it difficult to target and eliminate them entirely without harming healthy cells.
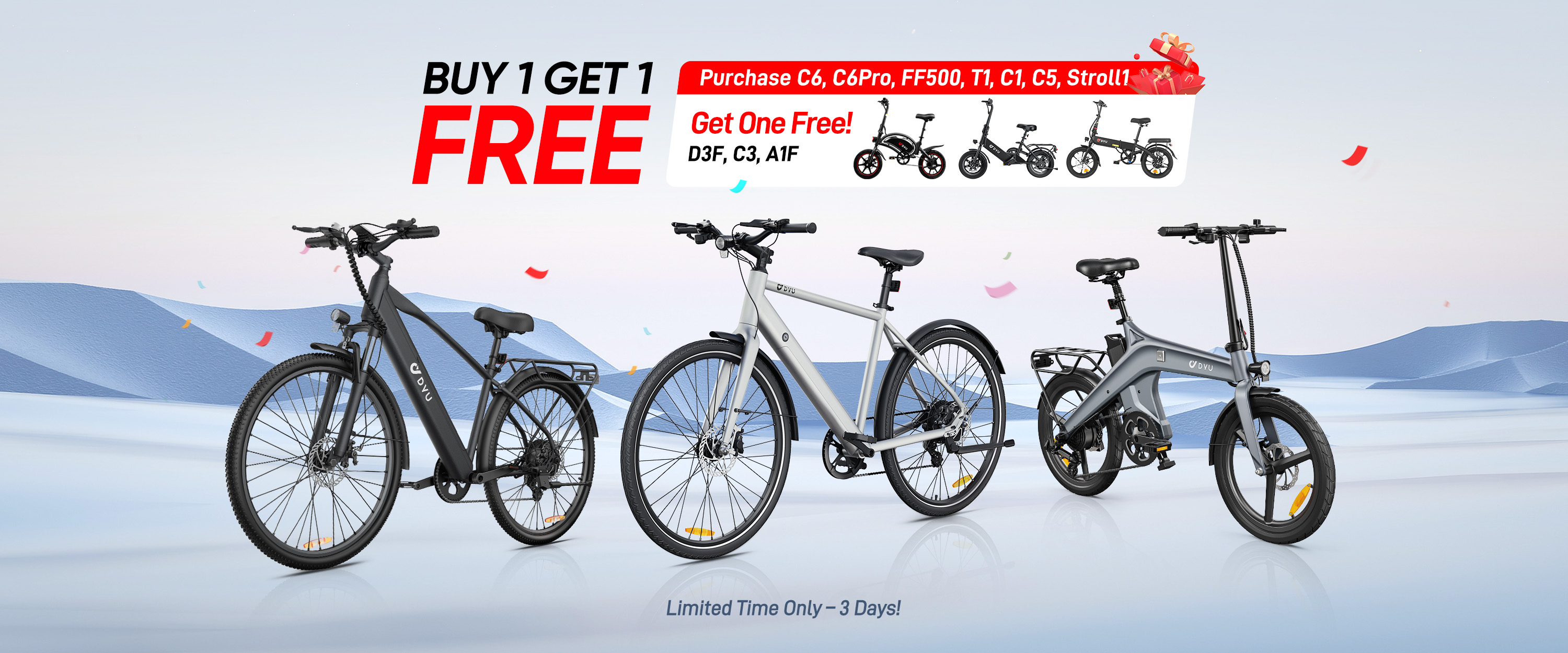
Cancer is difficult to cure for several key reasons:
- Cellular diversity: Cancer cells within a tumor can be genetically diverse, making uniform treatment challenging.
- Adaptability: Cancer cells can rapidly evolve and develop resistance to treatments.
- Similarity to normal cells: Cancer cells originate from normal cells, making it hard to target them without harming healthy tissue.
- Spread and metastasis: By the time cancer is detected, it may have already spread to other parts of the body.
- Complexity: Cancer involves multiple genetic and environmental factors, making it a highly complex disease.
- Immune evasion: Cancer cells can develop mechanisms to hide from or suppress the immune system.
- Heterogeneity between patients: Each person’s cancer can be unique, requiring personalized treatment approaches.

Cancer is Not a Single Disease
Cancer is a term that encompasses a wide variety of diseases, each with its own unique characteristics and behaviors. Here are some examples to illustrate this diversity:
Breast Cancer vs. Lung Cancer
- Breast Cancer: This type of cancer originates in the cells of the breast. It can be hormone receptor-positive, meaning it grows in response to hormones like estrogen or progesterone. Treatments often include hormone therapy, chemotherapy, radiation, and surgery.
- Lung Cancer: This cancer starts in the lungs and is often associated with smoking. It can be classified into small cell lung cancer (SCLC) and non-small cell lung cancer (NSCLC). Treatments may include targeted therapy, immunotherapy, chemotherapy, and surgery.
Despite both being cancers, the origins, behaviors, and treatments for breast cancer and lung cancer are quite different.
Leukemia vs. Melanoma
- Leukemia: This is a cancer of the blood and bone marrow. It leads to the production of abnormal white blood cells. Leukemia is treated with chemotherapy, radiation therapy, and sometimes stem cell transplants.
- Melanoma: This is a type of skin cancer that develops from melanocytes, the cells that produce pigment. It is often caused by excessive exposure to ultraviolet (UV) radiation from the sun. Treatments include surgery, immunotherapy, targeted therapy, and radiation.
Leukemia affects the blood and bone marrow, while melanoma affects the skin, highlighting the diverse nature of cancers.
Prostate Cancer vs. Pancreatic Cancer
- Prostate Cancer: This cancer occurs in the prostate gland in men. It is often slow-growing and may not require immediate treatment. Options include active surveillance, surgery, radiation therapy, and hormone therapy.
- Pancreatic Cancer: This is a highly aggressive cancer that starts in the pancreas. It is often diagnosed at a late stage and has a poor prognosis. Treatments include surgery, chemotherapy, and targeted therapy
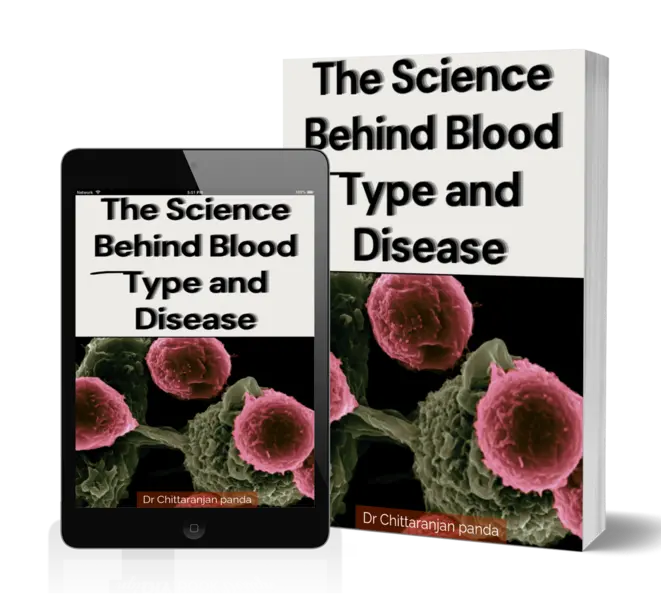
Genetic Mutations and Variability
Genetic mutations are changes in the DNA sequence of a cell. These mutations can lead to significant variability in how cancers develop and respond to treatment. Here are some examples to illustrate this concept:
BRCA1 and BRCA2 Mutations in Breast Cancer
- BRCA1 and BRCA2 Genes: Mutations in these genes significantly increase the risk of developing breast and ovarian cancers. These genes normally help repair DNA damage, but when mutated, they fail to do so, leading to cancer development.
- Example: Angelina Jolie famously underwent a preventive double mastectomy after discovering she carried a BRCA1 mutation, which gave her an estimated 87% risk of breast cancer1.
EGFR Mutations in Lung Cancer
- EGFR Gene: Mutations in the Epidermal Growth Factor Receptor (EGFR) gene are common in non-small cell lung cancer (NSCLC). These mutations cause cells to grow and divide uncontrollably.
- Example: Patients with EGFR mutations often respond well to targeted therapies like gefitinib or erlotinib, which specifically inhibit the EGFR protein2.
KRAS Mutations in Colorectal Cancer
- KRAS Gene: Mutations in the KRAS gene are found in about 40% of colorectal cancers. These mutations lead to continuous cell growth and division.
- Example: KRAS mutations can make colorectal cancer resistant to certain targeted therapies, such as those targeting the EGFR pathway3.
TP53 Mutations in Various Cancers
- TP53 Gene: Known as the “guardian of the genome,” the TP53 gene helps prevent cancer by repairing DNA or initiating cell death if the damage is irreparable. Mutations in TP53 are found in many types of cancer, including breast, lung, and colorectal cancers.
- Example: A TP53 mutation can lead to a loss of this protective function, allowing cancer cells to grow and spread unchecked

Heterogeneity Within Tumors
Tumor heterogeneity refers to the presence of a diverse population of cancer cells within a single tumor. This diversity can occur at multiple levels, including genetic, phenotypic, and functional differences among the cells. Here are some examples to illustrate this concept:
Genetic Heterogeneity
- Example: In a single tumor, different regions may have distinct genetic mutations. For instance, one part of a lung tumor might have an EGFR mutation, while another part has a KRAS mutation. This genetic diversity can lead to varied responses to treatment within the same tumor.
- Phenotypic Heterogeneity
- Example: Cancer cells within a tumor can exhibit different physical characteristics, such as size, shape, and protein expression. In breast cancer, some cells might express high levels of hormone receptors (like estrogen receptors), while others do not. This can affect how the tumor responds to hormone therapy.
Functional Heterogeneity
- Example: Different cancer cells within a tumor can have varying abilities to grow, invade tissues, and resist treatment. In glioblastoma, a type of brain cancer, some cells might be highly invasive, spreading quickly to other parts of the brain, while others might be more resistant to chemotherapy.
Microenvironmental Heterogeneity
- Example: The tumor microenvironment, which includes surrounding blood vessels, immune cells, and other support cells, can vary within different regions of the tumor. In pancreatic cancer, some areas of the tumor might be well-supplied with blood, while others are hypoxic (low in oxygen). This can influence how different parts of the tumor respond to treatments like radiation therapy.
Impact on Treatment
The heterogeneity within tumors poses significant challenges for treatment:
- Resistance to Therapy: Different subpopulations of cancer cells may respond differently to the same treatment. For example, while chemotherapy might kill the majority of cancer cells, a small subset with specific mutations might survive and cause a relapse.
- Targeted Therapy Limitations: Targeted therapies are designed to attack specific genetic mutations. However, if a tumor has multiple mutations, a single targeted therapy might not be effective against all cancer cells.
- Adaptive Responses: Cancer cells can adapt to their environment and develop resistance mechanisms. For instance, if a tumor is treated with a drug that targets a specific pathway, cancer cells might activate alternative pathways to survive.
Resistance to Treatment
Cancer cells can develop resistance to treatments over time, making it challenging to achieve long-term remission. This resistance can occur through various mechanisms and can affect different types of cancer treatments, including chemotherapy, targeted therapy, and immunotherapy. Here are some examples to illustrate this concept:
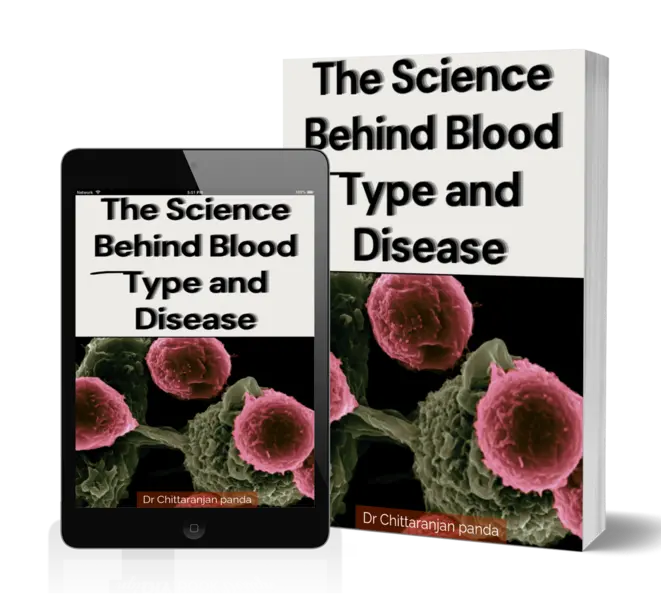
Chemotherapy Resistance
- Example: In ovarian cancer, patients often respond well to platinum-based chemotherapy initially. However, over time, the cancer cells can develop resistance, leading to a recurrence of the disease. This resistance can occur through several mechanisms, such as increased DNA repair capabilities of the cancer cells or changes in drug transport within the cells.
Targeted Therapy Resistance
- Example: In chronic myeloid leukemia (CML), the drug imatinib (Gleevec) targets the BCR-ABL fusion protein, which is responsible for the uncontrolled growth of leukemia cells. While imatinib is highly effective initially, some patients develop resistance due to additional mutations in the BCR-ABL gene. These mutations alter the protein’s structure, preventing imatinib from binding effectively.
Immunotherapy Resistance
- Example: In melanoma, immunotherapy drugs like pembrolizumab (Keytruda) work by enhancing the immune system’s ability to recognize and attack cancer cells. However, some melanoma cells can develop resistance by upregulating proteins that inhibit immune responses, such as PD-L1. This allows the cancer cells to evade detection and destruction by the immune system.
Hormone Therapy Resistance
- Example: In hormone receptor-positive breast cancer, treatments like tamoxifen block estrogen receptors to prevent cancer cell growth. Over time, some cancer cells can become resistant by mutating the estrogen receptor or activating alternative growth pathways that do not rely on estrogen.
Mechanisms of Resistance
Cancer cells can develop resistance through various mechanisms, including:
- Genetic Mutations: New mutations can alter the target of the therapy, making the treatment less effective.
- Drug Efflux: Cancer cells can increase the expression of proteins that pump drugs out of the cell, reducing the drug’s intracellular concentration.
- DNA Repair: Enhanced DNA repair mechanisms can allow cancer cells to survive despite the DNA-damaging effects of chemotherapy.
- Alternative Pathways: Cancer cells can activate alternative signaling pathways to bypass the blocked pathway targeted by the therapy.
Impact on Treatment
Resistance to treatment poses significant challenges for cancer therapy:
- Relapse: Even if a treatment is initially effective, resistance can lead to a relapse of the disease.
- Combination Therapies: To overcome resistance, doctors often use combination therapies that target multiple pathways simultaneously. However, this approach can increase the risk of side effects.
- Personalized Medicine: Understanding the specific mechanisms of resistance in individual patients can help tailor treatments to overcome resistance and improve outcomes.

Cancer’s Ability to Spread
Cancer’s ability to spread, known as metastasis, is one of the most challenging aspects of the disease. Metastasis occurs when cancer cells break away from the primary tumor and travel to other parts of the body, forming new tumors. Here are some examples and explanations to illustrate this process:
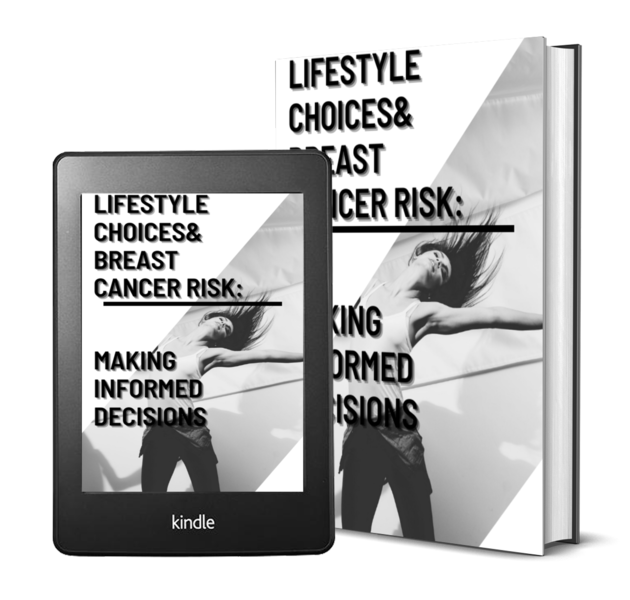
Breast Cancer Metastasis
- Example: Breast cancer cells can spread to various parts of the body, including the bones, liver, lungs, and brain. When breast cancer spreads to the bones, it can cause pain and fractures. If it spreads to the liver, it can lead to liver dysfunction and jaundice.
- Mechanism: Breast cancer cells can enter the bloodstream or lymphatic system, which acts as a highway for these cells to travel to distant organs. Once they reach a new site, they can establish a new tumor by adapting to the local environment.
Lung Cancer Metastasis
- Example: Lung cancer often spreads to the brain, bones, liver, and adrenal glands. Brain metastases can cause neurological symptoms such as headaches, seizures, and cognitive changes.
- Mechanism: Lung cancer cells can invade nearby blood vessels and travel through the bloodstream to distant organs. They can also spread through the lymphatic system, which drains fluid from tissues and returns it to the bloodstream.
Colorectal Cancer Metastasis
- Example: Colorectal cancer commonly spreads to the liver and lungs. Liver metastases can lead to liver enlargement, pain, and impaired liver function.
- Mechanism: Colorectal cancer cells can spread through the portal vein, which carries blood from the intestines to the liver. This direct connection makes the liver a common site for metastasis.
Prostate Cancer Metastasis
- Example: Prostate cancer frequently spreads to the bones, particularly the spine, pelvis, and ribs. Bone metastases can cause severe pain, fractures, and spinal cord compression.
- Mechanism: Prostate cancer cells can spread through the bloodstream or lymphatic system. They often target bones because the bone microenvironment provides factors that promote cancer cell growth.
Factors Influencing Metastasis
Several factors contribute to the ability of cancer cells to spread:
- Genetic Changes: Mutations in certain genes can enhance the ability of cancer cells to invade tissues and spread to distant sites.
- Tumor Microenvironment: The surrounding environment of the tumor, including blood vessels, immune cells, and support cells, can influence the ability of cancer cells to metastasize.
- Cell Adhesion: Cancer cells can lose their ability to stick to each other, making it easier for them to break away from the primary tumor and travel through the body.
- Angiogenesis: The formation of new blood vessels (angiogenesis) can provide cancer cells with the nutrients and oxygen they need to grow and spread.
Impact on Treatment
Metastasis significantly complicates cancer treatment:
- Multiple Sites: Treating cancer that has spread to multiple sites requires a more comprehensive approach, often involving systemic therapies like chemotherapy, targeted therapy, or immunotherapy.
- Resistance: Metastatic cancer cells can be more resistant to treatment compared to the primary tumor, making it harder to achieve remission.
- Prognosis: The presence of metastasis generally indicates a more advanced stage of cancer and is associated with a poorer prognosis.
Impact on the Immune System
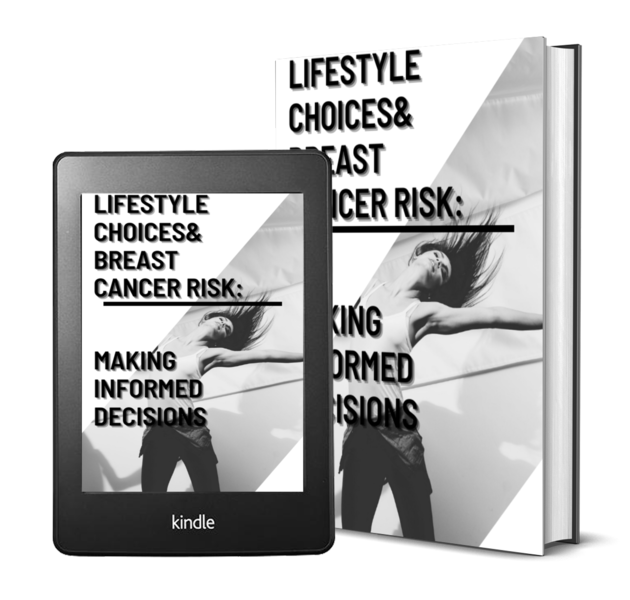
Cancer can significantly impact the immune system, both by evading immune detection and by actively suppressing immune responses. Here are some examples to illustrate how cancer interacts with the immune system:
Immune Evasion
- Example: Melanoma cells can express high levels of PD-L1, a protein that binds to the PD-1 receptor on T-cells (a type of immune cell). This interaction inhibits T-cell activity, allowing the cancer cells to evade immune detection and destruction.
- Mechanism: By expressing PD-L1, melanoma cells effectively “turn off” the immune response against them, making it difficult for the body to recognize and attack the cancer.
Immune Suppression
- Example: In ovarian cancer, the tumor microenvironment can be rich in regulatory T-cells (Tregs) and myeloid-derived suppressor cells (MDSCs). These cells suppress the activity of other immune cells that would normally attack the cancer.
- Mechanism: Tregs and MDSCs release cytokines and other factors that inhibit the function of cytotoxic T-cells and natural killer (NK) cells, which are crucial for targeting and killing cancer cells.
Chronic Inflammation
- Example: Chronic inflammation, such as that caused by hepatitis B or C infections, can lead to liver cancer. The persistent inflammatory environment promotes genetic mutations and cancer cell growth.
- Mechanism: Inflammation can cause DNA damage and create a microenvironment that supports cancer cell survival and proliferation. Immune cells that are constantly activated can also produce growth factors that aid in tumor development.
Immunotherapy and Immune Checkpoints
- Example: Immunotherapy drugs like pembrolizumab (Keytruda) and nivolumab (Opdivo) target immune checkpoints such as PD-1 and CTLA-4. These drugs block the inhibitory signals, allowing T-cells to attack cancer cells more effectively.
- Mechanism: By inhibiting the checkpoints, these drugs enhance the immune system’s ability to recognize and destroy cancer cells. This approach has shown success in treating cancers like melanoma, lung cancer, and renal cell carcinoma.
Impact on Treatment
The interaction between cancer and the immune system has significant implications for treatment:
- Immunotherapy: Leveraging the immune system to fight cancer has become a promising approach. Immunotherapies, such as checkpoint inhibitors and CAR-T cell therapy, aim to boost the body’s natural defenses against cancer.
- Combination Therapies: Combining immunotherapy with other treatments, like chemotherapy or radiation, can enhance the overall effectiveness. For example, radiation can increase the visibility of cancer cells to the immune system, making immunotherapy more effective.
- Personalized Medicine: Understanding the specific immune landscape of a patient’s tumor can help tailor immunotherapy treatments to achieve better outcomes.
Side Effects of Cancer Treatments
Cancer treatments, while effective at targeting cancer cells, can also affect healthy cells and tissues, leading to various side effects. Here are some examples of common cancer treatments and their associated side effects:
Chemotherapy
- Example: Chemotherapy drugs target rapidly dividing cells, which include both cancer cells and healthy cells like those in the bone marrow, digestive tract, and hair follicles.
- Side Effects:
- Bone Marrow Suppression: This can lead to a decrease in blood cells, causing anemia (fatigue), leukopenia (increased risk of infection), and thrombocytopenia (increased risk of bleeding).
- Gastrointestinal Issues: Nausea, vomiting, diarrhea, and mouth sores are common due to the impact on the digestive tract lining.
- Hair Loss: Damage to hair follicles can result in temporary hair loss.
Radiation Therapy
- Example: Radiation therapy uses high-energy particles or waves to destroy or damage cancer cells. It can also affect nearby healthy tissues.
- Side Effects:
- Skin Changes: Redness, blistering, and peeling of the skin in the treated area, similar to a sunburn.
- Fatigue: A common side effect due to the body’s response to radiation.
- Organ-Specific Effects: Depending on the area treated, radiation can cause specific side effects, such as difficulty swallowing (if the throat is treated) or urinary issues (if the pelvic area is treated).
Surgery
- Example: Surgical removal of tumors can be an effective treatment but comes with risks and side effects.
- Side Effects:
Hormone Therapy
- Example: Hormone therapy is used to treat cancers that are sensitive to hormones, such as breast and prostate cancer.
- Side Effects:
- Hot Flashes: Common in both men and women undergoing hormone therapy.
- Bone Thinning: Long-term use can lead to osteoporosis.
- Mood Changes: Hormone therapy can affect mood and emotional well-being.
Targeted Therapy
- Example: Targeted therapies are designed to specifically target cancer cells with certain genetic mutations.
- Side Effects:
Immunotherapy
- Example: Immunotherapy boosts the body’s immune system to fight cancer.
- Side Effects:
- Immune-Related Side Effects: Inflammation of healthy tissues, such as colitis (inflammation of the colon), pneumonitis (inflammation of the lungs), and hepatitis (inflammation of the liver).
- Flu-Like Symptoms: Fever, chills, and fatigue.
Managing Side Effects
Managing the side effects of cancer treatments is crucial for maintaining the quality of life for patients. Here are some strategies:
Monitoring and Adjustment: Regular monitoring of side effects allows healthcare providers to adjust treatment plans as needed to minimize adverse effects.
Medications: Anti-nausea drugs, pain relievers, and other medications can help manage specific side effects.
Supportive Care: Nutritional support, physical therapy, and counseling can help address the broader impacts of treatment.
Ongoing Research and Hope
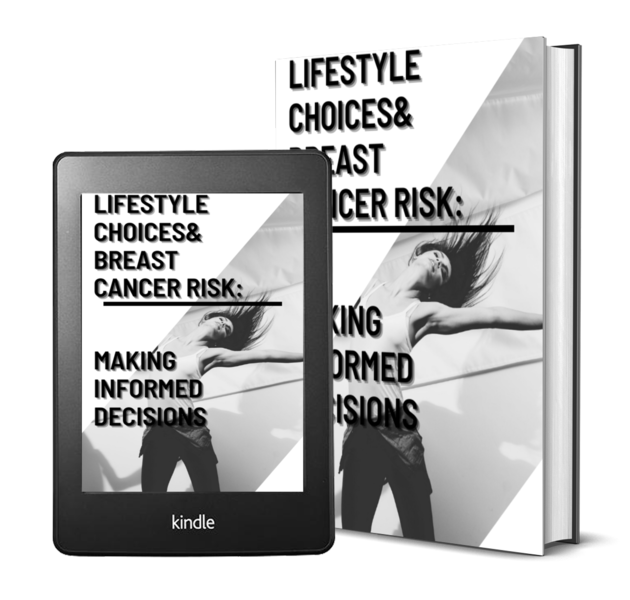
Despite the challenges in curing cancer, ongoing research is making significant strides in understanding the disease and developing new treatments. Here are some examples of promising areas of research and the hope they bring:
Targeted Therapy
- Example: Targeted therapies are designed to attack specific genetic mutations or proteins that drive cancer growth. For instance, drugs like trastuzumab (Herceptin) target the HER2 protein in certain breast cancers, significantly improving outcomes for patients with HER2-positive breast cancer.
- Hope: By focusing on the unique characteristics of cancer cells, targeted therapies can be more effective and have fewer side effects compared to traditional chemotherapy.
Immunotherapy
- Example: Immunotherapy harnesses the body’s immune system to fight cancer. Checkpoint inhibitors, such as pembrolizumab (Keytruda) and nivolumab (Opdivo), have shown success in treating cancers like melanoma, lung cancer, and renal cell carcinoma.
- Hope: Immunotherapy offers the potential for long-lasting responses and even cures in some cases, as it helps the immune system recognize and attack cancer cells more effectively.
CAR-T Cell Therapy
- Example: CAR-T cell therapy involves modifying a patient’s T-cells to express a receptor that targets cancer cells. This approach has been particularly successful in treating certain types of blood cancers, such as acute lymphoblastic leukemia (ALL) and diffuse large B-cell lymphoma (DLBCL).
- Hope: CAR-T cell therapy represents a personalized treatment approach that can lead to complete remissions in patients who have not responded to other treatments.
Liquid Biopsies
- Example: Liquid biopsies are blood tests that detect cancer-related genetic mutations and other biomarkers. They offer a non-invasive way to monitor cancer progression and response to treatment.
- Hope: Liquid biopsies can provide real-time insights into a patient’s cancer, allowing for more precise and timely adjustments to treatment plans.
Cancer Vaccines
- Example: Cancer vaccines aim to stimulate the immune system to recognize and attack cancer cells. The HPV vaccine, for instance, prevents infections with human papillomavirus, which can lead to cervical and other cancers.
- Hope: Preventive vaccines can reduce the incidence of certain cancers, while therapeutic vaccines are being developed to treat existing cancers by boosting the immune response.
Gene Editing
- Example: CRISPR-Cas9 is a gene-editing technology that allows scientists to precisely modify DNA. Researchers are exploring its use to correct genetic mutations that cause cancer or to enhance the immune system’s ability to fight cancer.
- Hope: Gene editing holds the promise of directly targeting the genetic causes of cancer, potentially leading to more effective and lasting treatments.